Human exposure to whole-body vibration should be evaluated using the method defined in ISO 2631-1. Whole-body vibration is applicable to motions transmitted from workplace machines and vehicles to the human body through a supporting surface. For health and safety evaluations, this is through the buttocks and feet of a seated person or the feet of a standing person.
When carrying out whole-body measurements, it is preferable to measure over the entire exposure time. If that is not possible or necessary, measurements should be made over periods of at least 20 minutes. Where short measurements are necessary, they should be at least three minutes long and should be repeated to achieve a total measurement time of more than 20 minutes. Longer measurements of 2 hours or more are preferable (half or full working day measurements are sometimes possible).
When assessing whole-body vibrations, acceleration should be picked up at the seat surface for a seated person or underneath the feet of a standing person. The accelerometer should be placed in a Seat Pad, which is preferably fixed to the floor or seat using tape or a strap to ensure that the accelerometer remains at the desired position and is able to withstand any position changes of the driver or operator of a machine. However, for correct results, the Seat Pad must be loaded during the measurement by the worker, who should stand or sit on the pad.
RMS vibration magnitude, Peak value, MTVV and VDV of the frequency-weighted acceleration should be measured simultaneously in all three directions, where Z-direction is always along the main body axis (i.e., for measurements at the feet and seat it is vertical to the seat and floor plane), the X-direction is aligned with the fore-and-aft motion and the Y-direction with a side-to-side motion.
In contrast to hand-arm vibration assessment, frequency weightings are different for X, Y, and Z-direction. In the context of health risk assessment, when measuring whole-body vibration at the feet and seat, ISO 2631-1 requires the use of Wk in the Z-direction, whereas Wd is used for the acceleration in the X and Y directions
Whole-body vibrations are measured with the help of the so-called seat sensor, where we need to install the triaxial sensor in the rubber adapter on which we sit. It is important that the z-axis is in a vertical direction since it is weighted differently than x and y.
Image 4: Seat pad sensorVibration should always be evaluated by measuring the weighted vibration acceleration. Crest factor determines the method for evaluation of the vibration. In the case of a high crest factor (greater than 9) to evaluate the vibration, running RMS or VDV should be used. Using the vector sum (total vibration value) is recommended for the evaluation of comfort and health risk assessment if the vibration in two or three axes are comparable.
For an assessment of occasional shocks and short-term vibration, the running RMS is used. In this case, the maximum value in the observation time is called as MTVV and is defined as the maximum instantaneous vibration magnitude. Vibration transmitted to the human body should be measured at a point between the body and the vibrating surface. In the case of a sitting person, this will be a seat, the seat back or the floor under feet.
Vibration should be measured according to the axis direction that begins at the point of human contact with the vibrating surface. The vertical axis (Z) should follow the orientation of the body, so the Z axis does not necessarily have to be vertical. The direction of the axis must be specified in the report.
The duration of the measurement shall be sufficient to ensure statistical accuracy and should take into account the typical vibration exposure. Measuring time should also be included in the report. The health of sitting persons is affected by vibrations in the frequency range of 0.5 Hz to 80 Hz. Measurements should be made using the appropriate frequency weightings: the Wk (Z-axis) and the Wd (axes X, Y). Because horizontal vibration is more harmful, the weighting coefficients should be 1.4 for the X, Y and 1 for the Z axis. Weighted RMS should be assessed in each of the 3 directions on the seat surface. In special cases, other weightings, such as Wc, Wf, We, Wj should be used.
Whole-body vibration should be evaluated on the basis of the result of the daily dose of A (8) expressed as equivalent frequency-weighted acceleration within eight hours, and calculated as the highest value of RMS or VDV determined in three axes X, Y, Z.
Image 5: Different measurement positions at whole-body vibration measurement
Based on the frequency-weighted acceleration signals, the daily vibration exposure is determined by calculating the exposure for each of the three axes separately and then selecting the highest of the three values. This necessitates an additional factor, ki, that must be applied to the measured vibration values. For the X and Y-direction, the factor is 1.4. For the Z-direction, the factor is 1.0:
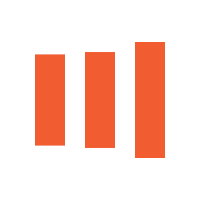
The maximum of these three values will then be the daily vibration exposure:
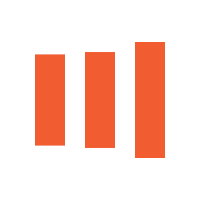
This is significantly different than the procedure used to determine hand-arm vibration exposure, where the three axes were combined to a single, total vibration value. However, according to ISO 2631-1, Section 6.5, a vibration total value may be used if no dominant axis of vibration can be found. The vibration total value for whole body vibration is calculated according to the following equation:
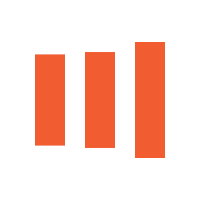
In some countries, different exposure limit values are given for different axes. Therefore, a paradox may occur such that, while the axis with the largest exposure value will not be found critical, another axis with a smaller exposure value will be above the limit for this axis. The report, based on the axis with the highest value, would not indicate a risk, even though the limit is violated for another axis.
If a worker is exposed to more than one source of vibration, the partial vibration exposure Aj, i(8), for each axis and operation i, is to be calculated first:
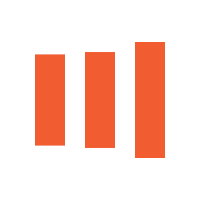
The partial vibration exposures are then added for each of the three axes separately, and the total daily vibration exposure is found as the maximum of these three sums:
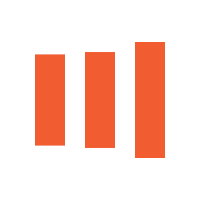
The total daily vibration dose, A(8), applies well if the vibration history is rather smooth, free of shocks or other sudden changes or peaks in the acceleration. However, when, for example, driving a vehicle over rough surfaces, such as found on construction sites and sandpits, shock like events may occur and an assessment based on RMS values may no longer be appropriate. The fourth power vibration dose value (VDV) has been developed to take such transients into account. Unlike RMS vibration magnitude, the measured VDV is a cumulative value and increases with the measurement time. It is, therefore, important for any measurement of VDV, to know the period over which the value was measured. Further, due to the fourth power, transients and peaks are given more weight in the integration. If the measurement time is shorter than the estimated exposure time, the measured VDV must be expanded to the actual exposure time:
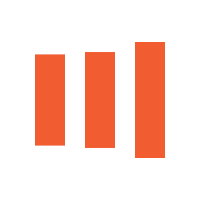
Where Tmeas is the measurement period and Texp is the full expected exposure time, and note again the k-factors (1.4, 1.4 and 1.0). Further, if a person is exposed to more than one vibration source, the total VDV is to be calculated from the partial vibration dose values for each axis:
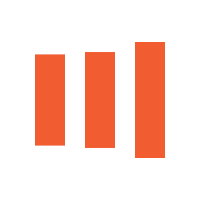
The highest of the three individual VDVs gives the daily VDV.
Another useful quantity when investigating human vibration with transients is the running RMS. It has a short integration time of 1 s and thus, is well suited to indicate the magnitude of short events. The so-called maximum transient vibration value (MTVV) represents the maximum running RMS value found over one measurement period.
ISO 2631-1 provides some guidelines concerning when it is recommended to consider VDV, running RMS and MTVV instead of the vibration magnitude, aw:
- If,
VDV should be considered in addition to RMS - If,
MTVV should be considered in addition to RMS - If,
VDV should be considered in addition to RMS
If one of these conditions is given, it indicates that the vibration history had peaks significantly above the general average vibration level.
The ratio between the Peak value and RMS vibration magnitude, the crest factor (CF), is considered to be a rather uncertain criterion because the peak may have occurred at a different time ranging from minutes to hours before or after the vibration event that determined the RMS.